Soil sensors play a crucial role in precision agriculture, environmental monitoring, and various ecological studies by providing real-time data on soil properties such as moisture, temperature, salinity, and nutrient levels. The accuracy and reliability of these sensors are paramount for making informed decisions that can impact crop yields, resource management, and environmental conservation. However, soil sensors often face challenges due to the complex and variable nature of soil environments. This article discusses the current advancements, challenges, and potential solutions for improving the accuracy and reliability of soil sensors. It explores material innovations, sensor design improvements, data processing techniques, and calibration methods that can enhance sensor performance under diverse soil conditions.
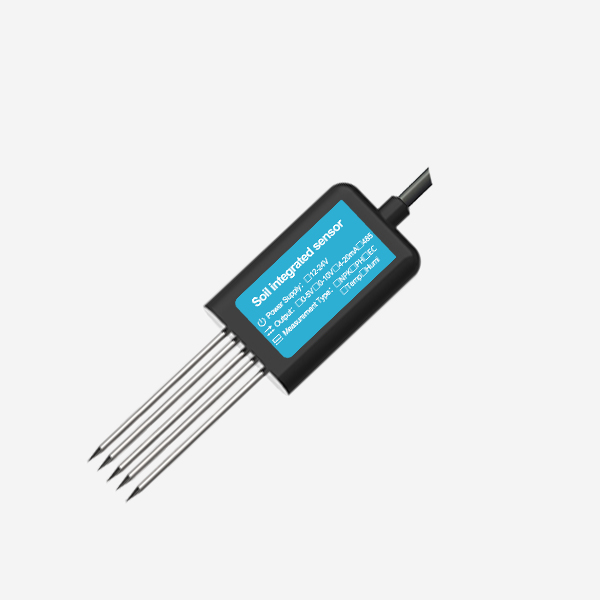
Introduction
Soil sensors are indispensable tools for modern agricultural practices and environmental sciences. They offer detailed insights into soil health, enabling farmers and researchers to optimize irrigation, fertilization, and soil management strategies. The growing demand for sustainable agriculture and efficient resource use underscores the importance of accurate and reliable soil sensor data. However, achieving this accuracy in practical field conditions is far from straightforward. Soils vary widely in composition, structure, and moisture content, which can significantly affect sensor performance.
This article aims to provide a comprehensive overview of the challenges faced by soil sensors and the strategies being developed to overcome them. By exploring advancements in sensor technology, data processing, and calibration techniques, we can gain a deeper understanding of how to improve the accuracy and reliability of soil sensors.
Challenges in Soil Sensor Accuracy and Reliability
Soil Variability
Soil variability is one of the most significant challenges in soil sensing. Soils differ widely in texture (sand, silt, clay content), structure (aggregation, porosity), organic matter content, and mineralogy. These variations can affect sensor readings in several ways:
- Electrical Conductivity: Differences in soil salinity and ion content can alter the electrical conductivity, influencing sensors that rely on this property, such as those measuring soil moisture or salinity.
- Temperature Sensitivity: Soil thermal properties vary, affecting how sensors respond to temperature changes.
- Physical Interference: Soil particles can clog sensor pores or interfere with sensing elements, leading to inaccurate readings.
Environmental Conditions
Environmental factors such as temperature, humidity, and soil compaction can also affect sensor performance. Temperature fluctuations can cause drift in sensor readings, while humidity can impact the electrical properties of soil and sensors. Soil compaction can alter soil porosity and moisture distribution, further complicating sensor measurements.
Sensor Limitations
Sensor design and technology also present limitations. Many soil sensors are designed for specific soil types or conditions, limiting their applicability across different environments. Additionally, sensor drift over time and exposure to harsh field conditions can degrade performance.
Advancements in Sensor Technology
Material Innovations
Recent advancements in materials science have led to the development of more durable and accurate sensors. New materials with improved chemical and physical stability can withstand harsh soil conditions and reduce drift over time. For example, ceramic-based sensors offer better resistance to corrosion and wear, while polymer-based composites can provide enhanced sensitivity and selectivity.
Sensor Design Improvements
Advancements in sensor design are also addressing some of the challenges posed by soil variability. Multi-sensor arrays and integrated systems that measure multiple soil properties simultaneously can provide a more comprehensive understanding of soil conditions. Additionally, sensors with adaptive algorithms that can compensate for environmental changes in real-time are being developed.
- Capacitive Sensors: These sensors measure soil moisture by sensing changes in capacitance caused by water content. Advancements in capacitive sensor design, such as improved electrode materials and geometries, have enhanced their accuracy and stability.
- Optical Sensors: Optical sensors use light absorption, reflection, or scattering to measure soil properties. Advances in spectrophotometry and fiber-optic technology have improved the precision of optical sensors, making them suitable for measuring soil nutrients and organic matter content.
- Electromagnetic Induction Sensors: These sensors measure soil electrical conductivity and can be used to estimate soil moisture and salinity. Recent developments in electromagnetic induction technology have led to sensors with higher resolution and deeper penetration depths.
Nanotechnology
Nanotechnology is emerging as a promising area for improving soil sensor accuracy and reliability. Nanomaterials, such as graphene and metal oxides, offer unique properties that can enhance sensor sensitivity, selectivity, and durability. Nanoscale sensors can also provide higher spatial resolution, allowing for more detailed measurements of soil properties.
Data Processing and Calibration Techniques
Advanced Data Processing
Advances in data processing techniques are crucial for extracting accurate and reliable information from soil sensor data. Machine learning and artificial intelligence algorithms can analyze complex soil data sets, identify patterns, and predict soil properties with high accuracy. These techniques can also be used to develop adaptive algorithms that compensate for environmental changes and sensor drift.
- Machine Learning Algorithms: Algorithms such as support vector machines, random forests, and neural networks can be trained on large datasets to predict soil properties based on sensor readings. These models can adapt to new data and improve over time.
- Data Fusion: Combining data from multiple sensors can provide a more comprehensive view of soil conditions. Data fusion techniques, such as Kalman filtering and Bayesian networks, can integrate information from different sensors to improve accuracy and reliability.
Calibration Methods
Calibration is a critical step in ensuring the accuracy of soil sensors. Traditional calibration methods, such as using reference samples or laboratory measurements, can be time-consuming and labor-intensive. However, recent advancements in calibration techniques are making this process more efficient and effective.
- In-situ Calibration: In-situ calibration methods involve calibrating sensors directly in the field using known soil properties. This approach reduces the need for laboratory measurements and can provide more representative calibration data.
- Remote Calibration: Remote calibration techniques use satellite or drone imagery to collect calibration data over large areas. This can be particularly useful for precision agriculture, where spatial variability in soil properties is significant.
- Automated Calibration Systems: Automated calibration systems can continuously monitor sensor performance and adjust calibration parameters in real-time. These systems can help maintain sensor accuracy over extended periods and reduce the need for manual calibration.
Practical Applications and Case Studies
Precision Agriculture
In precision agriculture, soil sensors are used to monitor crop health and optimize resource use. Improved sensor accuracy and reliability can lead to more efficient irrigation and fertilization practices, reducing water and nutrient waste. Case studies have shown that precision agriculture practices can increase crop yields and reduce environmental impact.
Environmental Monitoring
Soil sensors are also essential for environmental monitoring, particularly in areas affected by pollution or land degradation. Accurate measurements of soil properties can help assess the impact of contaminants and develop effective remediation strategies. Case studies have demonstrated the use of soil sensors in monitoring soil salinity, heavy metal contamination, and soil erosion.
Ecological Studies
Ecological studies often rely on soil sensors to understand the relationships between soil properties and ecosystem function. Improved sensor accuracy can provide more reliable data on soil nutrient cycling, microbial activity, and plant-soil interactions. Case studies in forest ecology, wetland restoration, and grassland management have highlighted the importance of accurate soil sensor data in ecological research.
Conclusion
Improving the accuracy and reliability of soil sensors is essential for advancing precision agriculture, environmental monitoring, and ecological studies. While soil variability, environmental conditions, and sensor limitations pose significant challenges, recent advancements in sensor technology, data processing, and calibration techniques offer promising solutions. By continuing to innovate and develop new methods for improving sensor performance, we can enhance our understanding of soil properties and make more informed decisions that benefit agriculture, the environment, and society.
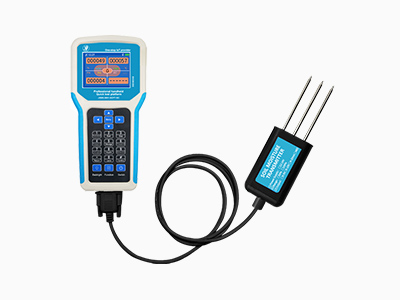
Future research should focus on integrating multidisciplinary approaches, such as materials science, data analytics, and environmental engineering, to develop more robust and adaptable soil sensor systems. Additionally, collaboration between researchers, farmers, and policymakers will be crucial for translating advancements in soil sensing technology into practical applications that promote sustainable development and resource conservation.